HOW DOES EROSION HAPPEN?WHAT IS WATER EROSION ? SNOW AS CAUSE OF SOIL EROSION.LANDSLIDES,MUDFLOWS,AND AVALANCHES ARE CALLED MASS WASTING.MASS WASTING IS SOMETIMES TRIGGERED BY A VOLCANIC ERUPTION OR BY AN EARTHQUAKE.
Rocks are gradually broken down into smaller pieces by the wind, rain, snow, and frost. For example, when water freezes in rock cracks, the water expands, slowly widening the cracks and fracturing the rock. The fragments of weathered rock are then blown away by the wind or carried away by water in the form of streams and rivers, ice in glaciers, or waves pounding coasts.Erosion is the wearing down and the carrying away of the Earth’s rock by the action of wind and moving water. Erosion happens fastest on steep hillsides after heavy rain, when LANDSLIDES sometimes strike.Weathering and erosion usually wear away landscapes fairly slowly. A landslide occurs when a huge mass of rock and soil suddenly breaks off a hillside, engulfing everything in its path. Landslides can cause great destruction and can even wipe out whole towns.Landslides often strike after heavy rain or snow has fallen. Loose soil and rocks begin to trickle downhill; then gravity takes over and the whole hillside slips away. Landslides are common on slopes where the vegetation has been removed by tree felling or farming. Without tree or plant roots to anchor the soil, heavy rain easily washes it away. Landslides, mudflows, and avalanches are called mass wasting. Mass wasting is sometimes triggered by a volcanic eruption or by an earthquake.Wind erodes the surface of a barren landmass in arid climates. Wind circulates and pushes particulates across wide bodies of water; a process known as deflation, which eventually leads to erosion. In addition, these particulates may collide with solid objects causing erosion by abrasion; a process known as ecological succession. Generally, wind erosion occurs in areas devoid of vegetation and rainfall to support growth, such as sand dune formation on a beach or desert.Geological landscapes may erode from one of five types of water erosion. Splash erosion occurs from the overall detachment and air transmission of soil particulates caused by excessive rainfall. Sheet erosion occurs from the detachment and movement of soil particulates by rainfall and sheet formation, instead of rill beds or water channels. The rainfall tears apart the sediment, which allows for soil particulates to re-fill the pores produced by the rainfall. Once the surface pores top off with soil aggregate, the water starts to lower off infiltration rates, thus causing runoff to take place.Rill erosion occurs with the formation of small, short-lived flow paths that function as sediment transference systems for sloping hills. Rills become active in areas where water erosion increases on disturbed landmasses. The flow depths of rills reach a few inches deep, which make them quite steep. As the rill beds change, the overall hydraulic flow dynamically changes the erosive rill patterns. The overall flow velocity, depth, width, slope, and detachment rate effect the formation of each rill bed. The flowing water enables the detachment of more sediment. If the overall rainfall rates exceed the soil’s infiltration mechanism, then it may actually result in the increase of more erosion than the rainfall’s impact.Gully erosion occurs as water flows down narrow channels after rainstorms and melting snow. Unlike rill beds, gullies typically remain intact, despite the surrounding tillage operations. A gully ranges in varying depths, usually ranging around one or two feet up to as much as one hundred feet. A continuous, linear water flow causes stream erosion. Stream erosion extends downward, deepening existing valleys, and expands the valley into the mountainside. Stream erosion occurs vertically, and each valley has a cross section where the gradient remains relatively steep. The erosive activity transitions into lateral erosive activity, which expands the valley floor. The stream sediment becomes flattened, which leads to the deposition of sediment as the stream flows along its floor’s plain. Stream erosion occurs more intensely during flooding. Kolks, vortices, and rock-cut basins are formed as a result of environments heavily affected by stream erosion.Lastly, bank erosion occurs with the wearing away of a stream or river’s shoreline. Bank erosion differentiates from the wearing away of a watercourse bed, often known as a scour. Geologists insert metal rods into an existing stream or river bank to correctly mark the position of the bank’s surface.Wind erosion produces yellowish sediment called loess. Loess has a porous, brittle, finely grained composition. It usually covers hundreds of square miles, and may measure up to tens of meters in overall thickness. Loess may stand in steep or vertical faces. It develops into highly rich soils, and may become a rich source for agricultural production if exposed to adequate weather conditions. Due to its geologically unstable nature, loess deposits erode at a rapid pace. As a result, farmers plant windbreaks to reduce the overall wind erosion of loess.Ice erosion occurs in one of two forms, the movement of glaciers, or thawing processes. In the latter formation, water inside pores and rock fractures expand, which causes further cracking. Glaciers erode through one of three different processes, including abrasion, plucking, and thrusting. Debris caught in the basal brushes along the bed, which polishes and gouges the rocks underneath. Glaciers also cause bedrock to fall off during the plucking phase. In addition, glaciers freeze and then move forward, which dislodges the sediments at the glacier’s base. The latter method produces thousands of lake basins that lie across the edge of the Canadian Shield. All of these combined processes form moraines, drumlins, kames, moulins, and glacial erratics, especially at the glacier retreat.Extreme cold weather temperatures cause trapped water particles to expand in its cracks, which breaks the rock into several pieces. This senior care leads to gravity erosion, particularly on steep slopes, and the formation of scree at the bottom of a mountainside. Morning thaws can present structural problems for roads alongside mountain cliffs. Additionally, trapped water in the wedge of a rock causes fissures, which eventually breaks down the rock.Spring thawing of snow can cause soil erosion. Its intensity is given mainly by water amount of snow, melting speed and soil infiltration capacity. In some regions of the Czech Republic, especially in slope areas with high amount of snow the early spring erosion can be significant factor of soil degradation. The paper introduces a relevant method to assess erosive potential of snow cover. The case study of its determination was carried out for long-term period on the base of available and comprehensive data of Czech Hydrometeorological Institute. Applied data were i) depth of snow cover, ii) water amount of snow, iii) depth of soil freezing.ALONG with his summary of publishe d data about the hardness of ice and d escriptions of wind-eroded features spatially associated with glaciation, Teichert concluded "There is thus no doubt that snow at low temperatures possesses the physical properties required for corrasion effects even on hard rocks". His "Iow temperature" requirem ent was base d on the fact that the hardness of ice increases with d ec reasing t emperature-for example, to 4 at -44°C and ::::::6 a t - 78.5 ° C (for this latter figure see Blackwelder ( 1939, p . 61» . Calculations made during our study of ventifacts indicate that the relative hardnesses of the missile and the targe t are not, as such, responsible for whether th ere is or is not erosion. Rather the kinetic energy of the missile and the bond strength of the target material d e termine the result. This, of course, means that hitting a relatively hard materi a l target with a rela -tive ly soft material projectile could r esult in breakage of the former as well as of t he latter. The main controls would b e the e ffective mass of the missile ("effective " rather than actual b ecaus e of diffe rences in shapes and sizes of c ollision surfaces) , the velocity of the missile, angular relationships at impact, and certain physical properties of the materials involved. R ecently, I have completed exp eriments that show the reported calculations do obtain. Among th e experiments was one in which snow was blown against cleavage blocks of calcite , fluorite and p ericlase ). The snow had irregularly shaped silt-sized particles of naturally agglomerated ice crystals. The temperature ranged between -10 and -2SoC which indica tes that the ice had a hardness ranging b etween approximately 2 and 3!. The wind velocities were from 14 to 23 m.p.h. Abrasion of the minerals used as targets was readily apparent within a few minutes. Breakage of pieces from corne rs and ed ges of the targets along with pitting of the fluorite were the obvious macro-scopic wind-blast features. These and microscopic features-all similar to those known to have b een formed by wind-blown dust and fine silt-will b e d escribed in a forthcoming paper. Although the experimental results support Teichert's conclusion tha t rocks can be abraded by wind-blown snow, it does not support his implication that such erosion occurs only a t temperatures below which th e hardness of snow (ice) is equal to or greate r than the h ardnesses of the abraded constituents of the target rocks. Instead , it shows that wind-blown, relatively soft snow may be an effective tool for rock abrasion. In addition, the experiment indicates that such snow projectiles may b e effective tools even when impell e d by only moderate winds-that is , the missile fragm ents do not need to have velocities generally designate d as of gale or hurricane strength. Briefly stated, the experiment shows that particles of snow blown at moderate wind speeds may abrade rocks. This possibility of erosion by wind-blown snow should b e kept in mind b y geomorpho-logists, glaciologists, paleoclimatologists and others wh o study and interpre t occurrences of ventifacts and wind-eroded bedrock. There are places where this phenomenon is most likely to cause problems, especially in flat and bare areas or dry and sandy soils. However, to a greater or lesser extent, we all suffer from the air pollution this event provokes.
Natural causes of air pollution: Wind Erosion.The soil erosion,that efforts have been made to contain the effects of the phenomenon in the country, as well as its social, psychological, economic and environmental impact.People in many areas aren’t conscious that wind erosion is a serious environmental problem and one of the most important natural causes of air pollution. There are places where this phenomenon is most likely to cause problems, especially in flat and bare areas or dry and sandy soils. However, to a greater or lesser extent, we all suffer from the air pollution this event provokes.
Soil erosion is a naturally occurring process that affects all landforms. In agriculture, soil erosion refers to the wearing away of a field's topsoil by the natural physical forces of water or through forces associated with farming activities such as tillage.Erosion, whether it is by water, wind or tillage, involves three distinct actions – soil detachment, movement and deposition. Topsoil, which is high in organic matter, fertility and soil life, is relocated elsewhere "on-site" where it builds up over time or is carried "off-site" where it fills in drainage channels. Soil erosion reduces cropland productivity and contributes to the pollution of adjacent watercourses, wetlands and lakes.Soil erosion can be a slow process that continues relatively unnoticed or can occur at an alarming rate, causing serious loss of topsoil. Soil compaction, low organic matter, loss of soil structure, poor internal drainage, salinisation and soil acidity problems are other serious soil degradation conditions that can accelerate the soil erosion process.This Factsheet looks at the causes and effects of water, wind and tillage erosion on agricultural land.Surface water runoff occurs whenever there is excess water on a slope that cannot be absorbed into the soil or is trapped on the surface. Reduced infiltration due to soil compaction, crusting or freezing increases the runoff. Runoff from agricultural land is greatest during spring months when the soils are typically saturated, snow is melting and vegetative cover is minimal.Soil erodibility is an estimate of the ability of soils to resist erosion, based on the physical characteristics of each soil. Texture is the principal characteristic affecting erodibility, but structure, organic matter and permeability also contribute. Generally, soils with faster infiltration rates, higher levels of organic matter and improved soil structure have a greater resistance to erosion. Sand, sandy loam and loam-textured soils tend to be less erodible than silt, very fine sand and certain clay-textured soils.Tillage and cropping practices that reduce soil organic matter levels, cause poor soil structure, or result in soil compaction, contribute to increases in soil erodibility. As an example, compacted subsurface soil layers can decrease infiltration and increase runoff. The formation of a soil crust, which tends to "seal" the surface, also decreases infiltration. On some sites, a soil crust might decrease the amount of soil loss from raindrop impact and splash; however, a corresponding increase in the amount of runoff water can contribute to more serious erosion problems.Past erosion also has an effect on a soil's erodibility. Many exposed subsurface soils on eroded sites tend to be more erodible than the original soils were because of their poorer structure and lower organic matter. The lower nutrient levels often associated with subsoils contribute to lower crop yields and generally poorer crop cover, which in turn provides less crop protection for the soil.Common all over the world, glaciated valleys are probably the most readily visible glacial landform. Similar to fjords, they are trough-shaped, often with steep near-vertical cliffs where entire mountainsides were scoured by glacial movement. One of the most striking examples of glaciated valleys can be seen in Yosemite National Park, where glaciers literally sheared away mountainsides, creating deep valleys with vertical walls.Fjords, such as those in Norway, are long, narrow coastal valleys that were originally carved out by glaciers. They are often “U-shaped,” with steep sides and rounded bottoms, giving them a trough-like appearance. Once the glaciers receded, seawater covered the floor of the glacial trough to create fjords.The famous Matterhorn in Switzerland displays three types of glacial erosion: Cirques are created when glaciers erode the mountainside, scouring into it and creating rounded hollows with steep uphill faces, shaped like tilted bowls. A cirque is often more visible after the glacier melts away and leaves the bowl-shaped landform behind.Arêtes are jagged, narrow ridges created where the back walls of two glaciers meet, eroding the ridge on both sides.Horns are created when several cirque glaciers erode a mountain until all that is left is a steep, pointed peak with sharp, ridge-like arêtes leading up to the top.Fjords, glaciated valleys, and horns are all erosional types of landforms, created when a glacier cuts away at the landscape. Other types of glacial landforms are created by the features and sediments left behind after a glacier retreats.When glaciers retreat, they often deposit large mounds of till: gravel, small rocks, sand, and mud. It is made from the rock and soil that was ground up beneath the glacier as it moved.Material a glacier picks up or pushes as it moves forms moraines along the surface and sides of the glacier. As a glacier retreats, the ice literally melts away from underneath the moraines, so they leave long, narrow ridges that show where the glacier used to be. Glaciers do not always leave moraines behind, however, because sometimes the glacier’s own meltwater washes the material away.Streams flowing from glaciers often carry some of the rock and soil debris out with them. These streams deposit the debris as they flow. Consequently, after many years, small steep-sided mounds of soil and gravel begin to form adjacent to the glacier, called kames.Kettle lakes form when a piece of glacier ice breaks off and becomes buried by glacial till or moraine deposits. Over time, the ice melts, leaving a small depression in the land, filled with water. Kettle lakes are usually very small, more like ponds than lakes.Glaciers leave behind anything they pick up along the way, and sometimes this includes huge rocks. Called glacial erratics or erratic boulders, these rocks might seem a little out of place, which is true, because glaciers have literally moved them far away from their source before melting out from underneath them.Drumlins are long, tear-drop-shaped sedimentary formations. What caused drumlins to form is poorly understood, but scientists believe that they were created subglacially as the ice sheets moved across the landscape during the various ice ages. Theories suggest that drumlins might have been formed as glaciers scraped up sediment from the underlying ground surface, or from erosion or deposition of sediment by glacial meltwater, or some combination of these processes. Because the till, sand, and gravel that form drumlins are deposited and shaped by glacier movement, all drumlins created by a particular glacier face the same direction, running parallel to the glacier's flow. Often, hundreds to thousands of drumlins are found in one place, looking very much like whale backs when seen from above.Aeolian erosion develops through two principal processes: deflation (removal of loosened material and its transport as fine grains in atmospheric suspension) and abrasion (mechanical wear of coherent material). The relative significance of each of these processes appears to be a function of the properties of surface materials and the availability of abrasive particles. The landforms that result from aeolian erosion include ventifacts, ridge and swale systems, yardangs, desert depressions, and inverted relief.The widespread occurrence of water erosion combined with the severity of on-site and off-site impacts have made water erosion the focus of soil conservation efforts.The rate and magnitude of soil erosion by water is controlled by the following factors:Rainfall and Runoff.The greater the intensity and duration of a rainstorm, the higher the erosion potential. The impact of raindrops on the soil surface can break down soil aggregates and disperse the aggregate material. Lighter aggregate materials such as very fine sand, silt, clay and organic matter are easily removed by the raindrop splash and runoff water; greater raindrop energy or runoff amounts are required to move larger sand and gravel particles.Soil movement by rainfall (raindrop splash) is usually greatest and most noticeable during short-duration, high-intensity thunderstorms. Although the erosion caused by long-lasting and less-intense storms is not usually as spectacular or noticeable as that produced during thunderstorms, the amount of soil loss can be significant, especially when compounded over time.
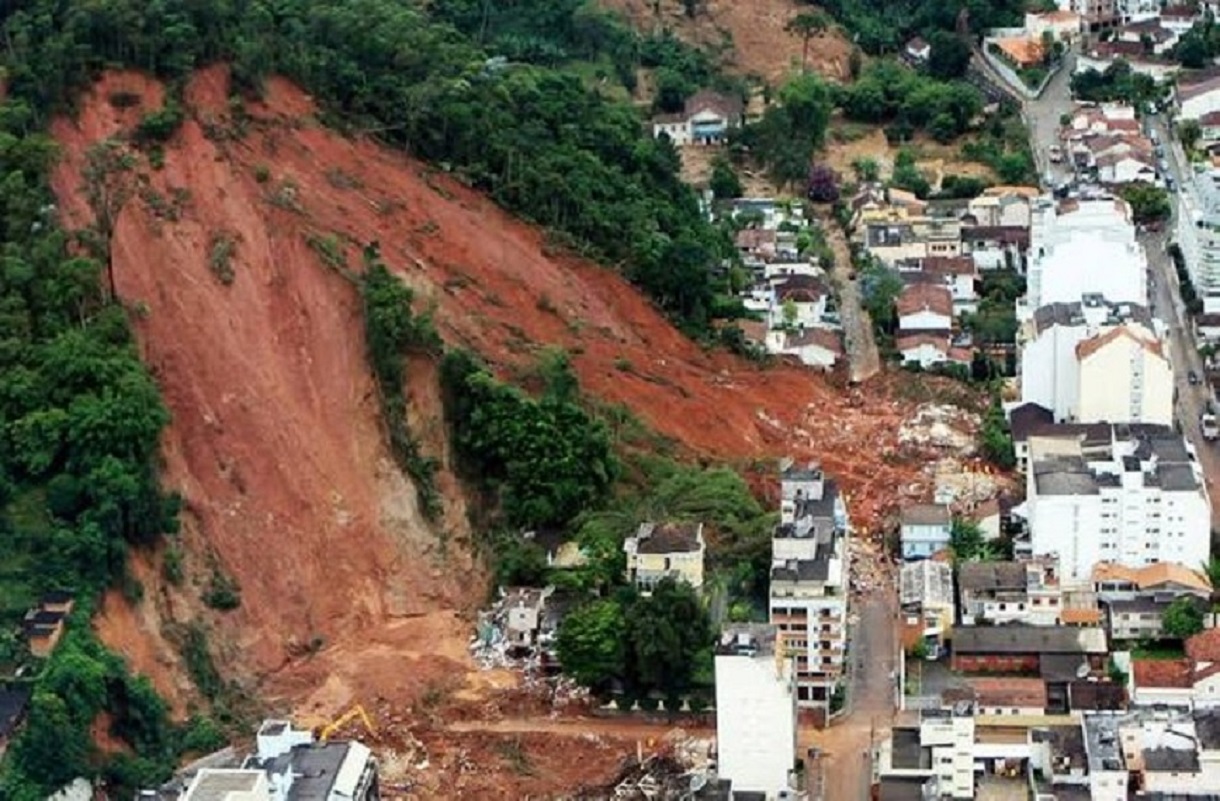
Rockslides are a type of landslide where there's a rapid downslope movement of newly detached segments of bedrock. Some causes of rockslides are cracking of the ground at the crown of the slide, or bulging of the ground surface above the toe of the rupture surface, natural weaknesses of rock, coal mining, and earthquakes.Cracking of the ground or bulging of the ground surface:displacement of rocks can weaken the rock easily triggering a rockslide.Natural weaknesses: Some rocks have surfaces of weakness separating the layers of sediment and where the rocks were formed. As erosion occurs through the mountains by rivers, glaciers, rain, wind, etc. the surfaces become exposed creating the possibility of rockslides occuring.Coal mining: human destruction of the land leaves the ground surface susceptible to rockslides.Earthquakes: the violent, intense shaking of the land can trigger a rockslide.
Erosion rate is highly variable along the Esposende municipality coast in relation with the morphology, the geological and hydrological context, the lithology of each coastal stretches, (Loureiro, 2006) as well as with the coast defense structures. The main observed effects of the erosion are a retreat of coastline, changes in beaches profiles, destruction of sandy beaches and dunes, land losses, damages and destruction of buildings and infrastructures, and indirectly an increase of the marine submersion hazard. The forcing factors of local erosion are the littoral drift and waves (erosive crisis during storms), but the main cause of the long-term erosion is due to a regional negative sedimentary budget and a weak physical resilience due to lack of sediment stock in the shoreface.The sandy beaches are generally affected by seasonal phases of erosion during winter (due to wave action during storms) and aggradation during summer. In Esposende coast, the lack of sediment stock in the shoreface does not allow the reconstruction of the profile range during summer. The existence of littoral drift can lead to a long shore transport of sediment along the coast. In some coastal stretches, the sedimentary budget (at the pluriannual scale) is globally positive inducing aggradation, on other coastal stretches; the sedimentary budget is null inducing stability. In the case of negative sedimentary budget which is the most common, the coast is in erosion.Thus, at the annual scale, erosion can be described as an oscillatory process around an average position of the coastline, with erosional events which can be “crisis erosional event” followed by a relaxation phase with sedimentary aggradation which tends to restore position of the coastline which prevails before erosion crisis. The space of oscillations can be presented as an uncertainty strip around a medium coastline position. This uncertainty strip can represent at a short time scale the area exposed to the erosion hazard (Crisis event: short-term erosional event with coastline retreat during a storm).Coastal erosion, in itself, is a reversible process at some timescales; the accretion phase can occur after erosion phase and lead to a coastline recovery. The physical resilience of a coastal strip is linked to the stock of sand and to the availability of this sand offshore and backshore for same conditions. Thus, the role of the sand dunes is of a primary importance to the resilience capacity of the coast.However, even if coastal erosion is reversible, the effect of coastal erosion on assets is irreversible. The buildings, roads, other infrastructures, natural habitats, etc. located on eroded lands are irremediably destroyed, lands and soils are definitely lost.Hence, in this approach, the fragility of assets to coastal erosion is assumed as Boolean an asset which is affected by the coastal erosion is definitely destroyed and damaged and unusable. Thus, we can consider that the fragility of assets (e.g., buildings, networks, roads) is a Boolean function with only two damage states.Therefore, the return period is a concept not adapted to the coastal erosion hazard. Instead, for a specific location we propose the concept and use of the time expectancy. For the application to the Esposende site, we chose a 30 years time expectancy consistent to the duration of the planning landscape projects. Noteworthy, in the frame of the MOVE project we have not taken into account the climate change (e.g., sea level rise, storm surge) which is an important component potentially acting on erosion processes.In order to locate the location of the 30 years time expectancy line, we have determined the erosion rates for each homogeneous coastal segment using data from Loureiro (2006). The analysis of the cross-shore profiles shows that the rate at each point can vary in time. In Esposende, the duration of recorded data concerning the evolution of the coastline due to erosion is too short to determine an average accurate and reliable rate.Glacial erosion rates are a major concern for geohazard management because erosion of cirques and troughs sets the base level for processes acting on adjacent slopes. Notably, the oversteepening of valley sides by glacial erosion leaves a legacy of slope instability that is itself a powerful agent of landscape modification and a concern for geohazard management .The potential for soil erosion increases if the soil has no or very little vegetative cover of plants and/or crop residues. Plant and residue cover protects the soil from raindrop impact and splash, tends to slow down the movement of runoff water and allows excess surface water to infiltrate.The erosion-reducing effectiveness of plant and/or crop residues depends on the type, extent and quantity of cover. Vegetation and residue combinations that completely cover the soil and intercept all falling raindrops at and close to the surface are the most efficient in controlling soil erosion (e.g., forests, permanent grasses). Partially incorporated residues and residual roots are also important as these provide channels that allow surface water to move into the soil.The effectiveness of any protective cover also depends on how much protection is available at various periods during the year, relative to the amount of erosive rainfall that falls during these periods. Crops that provide a full protective cover for a major portion of the year (e.g., alfalfa or winter cover crops) can reduce erosion much more than can crops that leave the soil bare for a longer period of time (e.g., row crops), particularly during periods of highly erosive rainfall such as spring and summer. Crop management systems that favour contour farming and strip-cropping techniques can further reduce the amount of erosion. To reduce most of the erosion on annual row-crop land, leave a residue cover greater than 30% after harvest and over the winter months, or inter-seed a cover crop.A lack of windbreaks (trees, shrubs, crop residue, etc.) allows the wind to put soil particles into motion for greater distances, thus increasing abrasion and soil erosion. Knolls and hilltops are usually exposed and suffer the most.he lack of permanent vegetative cover in certain locations results in extensive wind erosion. Loose, dry, bare soil is the most susceptible; however, crops that produce low levels of residue (e.g., soybeans and many vegetable crops) may not provide enough resistance. In severe cases, even crops that produce a lot of residue may not protect the soil.The most effective protective vegetative cover consists of a cover crop with an adequate network of living windbreaks in combination with good tillage, residue management and crop selection.Wind erosion damages crops through sandblasting of young seedlings or transplants, burial of plants or seed, and exposure of seed. Crops are ruined, resulting in costly delays and making reseeding necessary. Plants damaged by sandblasting are vulnerable to the entry of disease with a resulting decrease in yield, loss of quality and market value. Also, wind erosion can create adverse operating conditions, preventing timely field activities.Soil drifting is a fertility-depleting process that can lead to poor crop growth and yield reductions in areas of fields where wind erosion is a recurring problem. Continual drifting of an area gradually causes a textural change in the soil. Loss of fine sand, silt, clay and organic particles from sandy soils serves to lower the moisture-holding capacity of the soil. This increases the erodibility of the soil and compounds the problem.The removal of wind-blown soils from fence rows, constructed drainage channels and roads, and from around buildings is a costly process. Also, soil nutrients and surface-applied chemicals can be carried along with the soil particles, contributing to off-site impacts. In addition, blowing dust can affect human health and create public safety hazards.